Validating greenhouse gas monitoring capabilities and satellite sensor interoperability
Task 3.1 within Theme 3 ‘Validating Carbon stocktake: GHG emissions and biosphere’ of MetEOC-4 is applying robust metrological methods developed in previous studies to address satellite-derived measures of atmospheric greenhouse gas concentrations. In this update, we outline details of some of the groundwork involved in validating gas monitoring capabilities and sensor interoperability for coming earth observation missions.
While the main driver of changes in atmospheric greenhouse gases (GHGs) over the past 200 years relate to direct emissions, the accumulation of these gases in the atmosphere has also been influenced by natural biological and chemical processes that cause ecosystems to act as net carbon sources or sinks.
A carbon sink is anything, natural or otherwise, that accumulates and stores carbon-containing chemical compounds for an indefinite period, thereby (for a time) removing carbon dioxide (CO2) from the atmosphere.
Levels of CO2 have always fluctuated to some degree, but the recent unprecedented rates of increase, due to anthropogenic combustion of hydrocarbons and factors such as deforestation, results in highly uncertain predicted trajectories of likely environmental response over timescales relevant to ‘net zero’ policy.
An uncertain path for GHG sources and sinks
The Technical Summary of the latest IPCC report on The Physical Science Basis of understanding of the climate system and climate change states ‘continued growth of atmospheric CO2 concentrations over the industrial era is unequivocally due to emissions from human activities’. While emphasising that burning hydrocarbons was the dominant factor, the report notes that other processes have had some influence, including in carbon sinks, that absorbed some of the CO2 that otherwise would have remained in the atmosphere.
For instance, over the decade 2010 to 2019, the report states that of all annual human-produced CO2 emissions 46% was accumulated in the atmosphere, 23% was taken up by the ocean and 31% was removed by terrestrial ecosystems.
However, nature’s response cannot be taken for granted, especially where conditions are changing at an unprecedented pace. For example, soil is an important carbon storage medium but changing land use or other factors can lead to this type of carbon absorber becoming a net emitter.
The IPCC expects levels of carbon uptake by such sinks to be influenced to varying degrees according to the emissions scenarios that actually occur. Land and ocean sinks could absorb more CO2 under high emissions scenarios than low emissions scenarios, but the fraction of emissions removed from the atmosphere by natural sinks would decrease with higher concentrations.
Seasonal and yearly variability of the responses of ocean and land sinks also suggests sensitivity of these sinks to climate change, to the extent that sinks could act as sources in some circumstances.
Earth system model forecasts of the trajectory of future CO2 emissions in the report show that the (hard to predict) uncertainties of future human contributions dominate overall uncertainty but uncertainties of carbon–climate feedbacks are significant.
The imprecise nature of current estimations is also illustrated by uncertainty analyses provided in the 2022 UK National Inventory Submissions to UNFCC, reporting estimated uncertainties in 1990 and 2020 figures for Land-Use, Land Use Change and Forestry of 20% for CO2, 40-45% for CH4, and 45-50% for N2O.
Such uncertainties have consequences for future policymaking, as shown by the IPCC AR5 projections for various CO2 emissions reduction scenarios (see below).
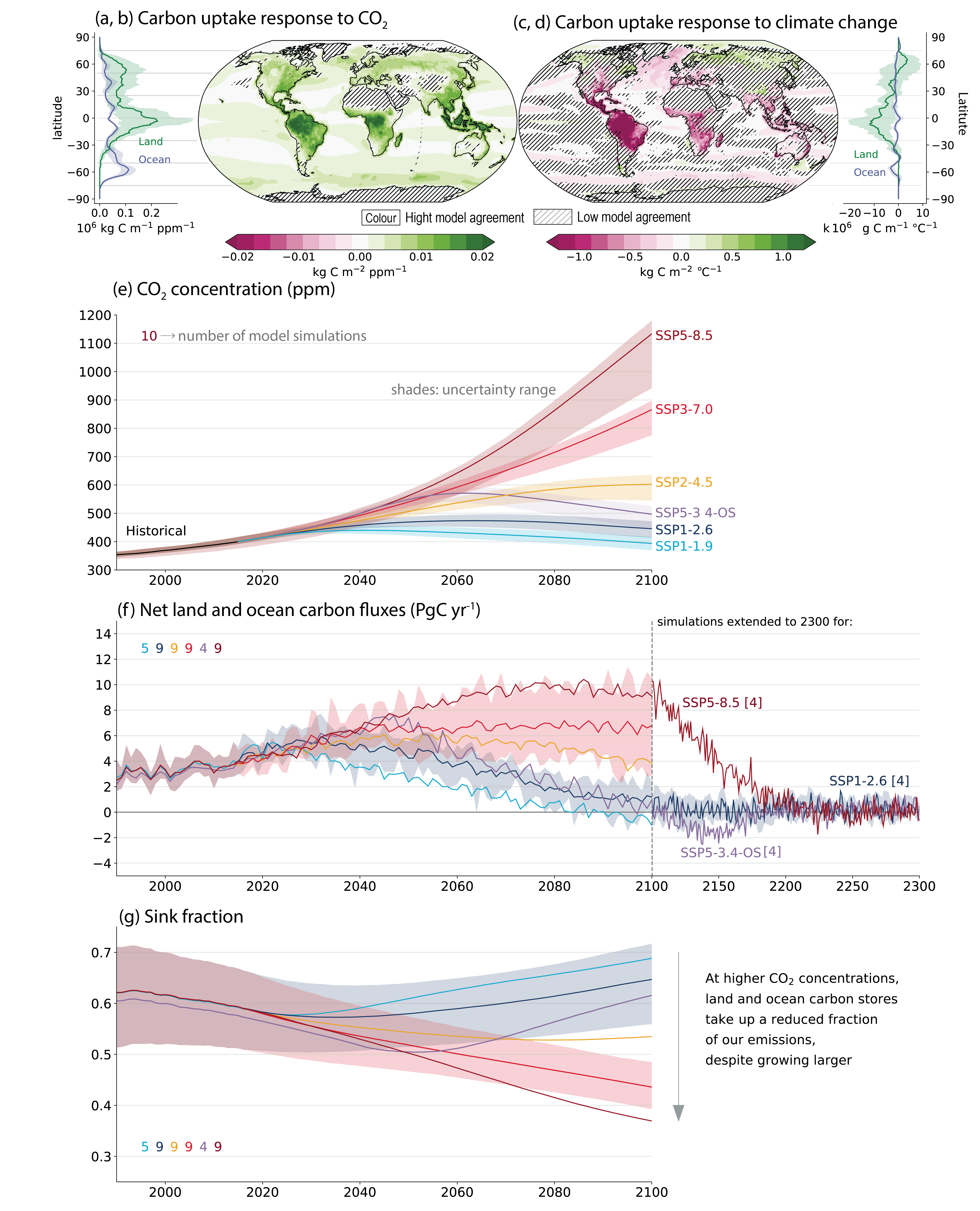
A clearer picture of future of GHG emissions trajectories could emerge, however, with improved quantification of the uncertainties associated with measuring sources and sinks of greenhouse gases.
A new generation of GHG monitoring missions
Earth observation missions are being developed to provide precise and actionable estimates of greenhouse gas emissions for various applications: at the continental scale for science purposes; at the country scale for national reporting to the UNFCCC; at the sub-national scale (states, cities, and regions) for planning; and point sources for direct monitoring, such as for large power plants.
Japan, United States and China have launched satellite missions to monitor greenhouse gases.
Currently in its final integration phase, the MICROCARB mission — led by the French space agency CNES and also funded by the national research agency ANR in partnership with the United Kingdom Space Agency (UKSA) — is expected to be launched in early 2024. Microcarb will provide information on vertical CO2 concentration columns for monitoring surface fluxes above land, atmosphere, oceans, and vegetation, and the first to use these to retrieve source and sink terms. Using a passive sounding technique, Microcarb’s spectrometer will measure solar luminance after reflection from the Earth’s surface, operating in four spectral bands (0.76 and 1.27 μm for O2 measurement, 1.6 and 2 μm for CO2 measurement). The mission will be able to measure atmospheric concentrations of CO2 globally to a high degree of precision (at about 1 ppm), imaging with a pixel size of 4.5 km x 9 km.
Another GHG monitoring capacity is the CO2 emissions Monitoring and Verification Support Capacity (CO2MVS), which from 2026 will form a major part of the EU’s Copernicus Atmosphere Monitoring Service (CAMS) operated by ECMWF.
Meteoc Theme 3 Task 1 — understanding uncertainties and providing insights to enhance emissions estimates
The objective of Task 3.1 of MetEOC-4 is to recommend suitable measurement and verification requirements for remote sensing of GHG amounts.
According to NPL’s Earth Observation & Climate group Science Area Lead Paul Green, “As part of the push to understand emissions reductions and climate reduction methods, new series of satellites such as Microcarb will quantify to higher spatial resolutions. These satellites will not just measure how much greenhouse gases are in the atmosphere, as could be done previously, but also detect the flux, to identify where it’s coming from”.
“Numerous assumptions exist in the concept of ‘Net Zero’. The contribution of this task is to understand the accuracy to which we can quantify emissions as coming from that country, that region, that power station, or also that wetland area or that area of deforestation. These satellite sensors will quantify both anthropogenic and natural sources of emissions, which will have implications for national and country reporting activities”, added Paul, who is leading this part of the project.
Using published data, reports, and assessments of anticipated sensor performance — an uncertainty framework will be developed, applying rigorous metrological principles to identify metrological gaps for GHG sensors.
A strategy for enhancing the metrology of such sensors will be devised, and a numerical model will explore the effects of uncertainty contributions. This model will be extended to provide a tool to analyse uncertainties for the interoperability of satellite sensors, addressing common algorithms, assumptions, and ancillary data that may bias individual sensor performance.
Then, after consulting the earth observation community, a roadmap of metrological requirements for enabling robust traceability of GHG sensors will be proposed, serving that community and the objectives of the Paris Agreement.
Taking a break from crunching the data, Paul explained further, “We want to understand how uncertainties propagate through the retrieval algorithms used for these missions”.
“Sensor technologies measure ratios of light at 1.6 and 2.2 microns, and then from retrieved CO2 column amounts. We’re working out whether the uncertainties being reported for those measurements are realistic.”
“A second layer of what we’re doing is putting one of these retrieval algorithms through a Fiduceo-like method to uncover gaps in understanding or overlooked assumptions. Then we can work out how to better quantify uncertainties in those gaps. That will help to combine data from multiple satellites, as different satellites use different measurement techniques.”
“We will then present a report recommending what needs to be done to create datasets from the satellites in a seamless, harmonised way”.
Powerful substances have big effects
The climate observation system envisaged by MetEOC offers the prospect of a more meaningful understanding of the effects of sources and sinks of greenhouse gas emissions.
With that in mind, Paul’s first task each morning is, naturally, loading up his French press with robust coffee grounds. A daily, if purely coincidental, reminder that (as Tweeted recently by WMO and NASA) small quantities of a powerful substance can have big effects.
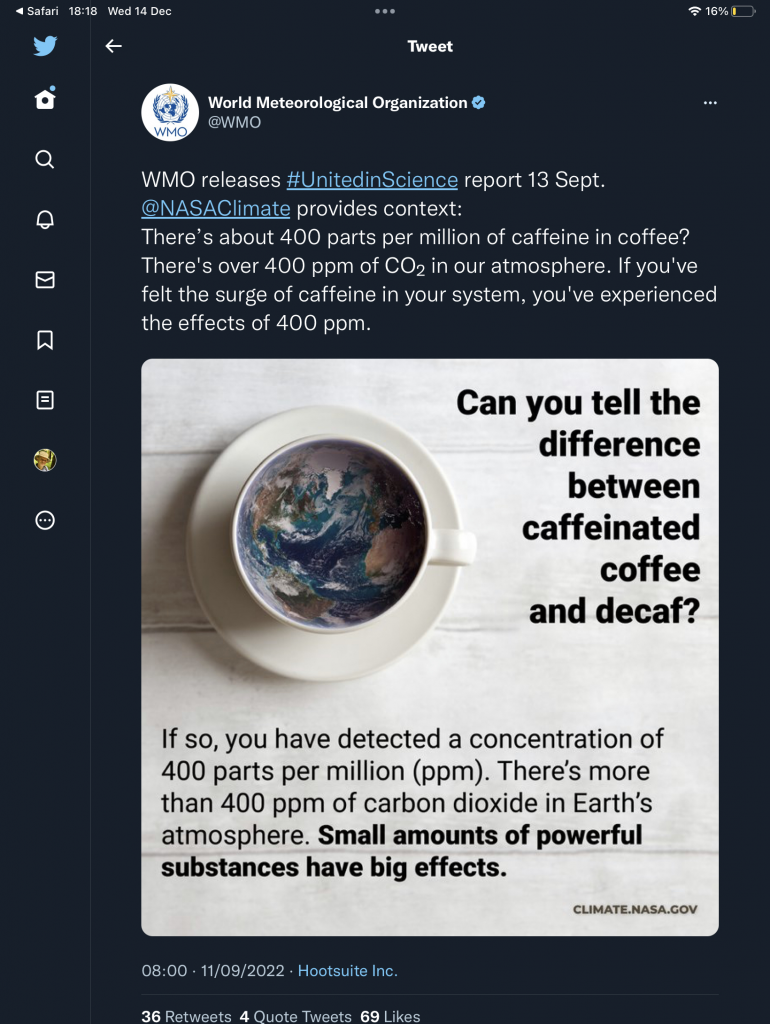
Near the conclusion of MetEOC-4, a roadmap of metrological requirements for ensuring traceable GHG sensors will be shared. The ultimate result of which could be fewer sleepless nights for the monitoring communities tasked with providing observations that support the objectives of the Paris Agreement.